Opening the Black Box of Dendritic Computing
How do nerve cells compute? This fundamental question drives LMU neurobiologists led by Andreas Herz. They have now presented a novel method to disentangle complex neural processes in a much more powerful way than was previously possible.
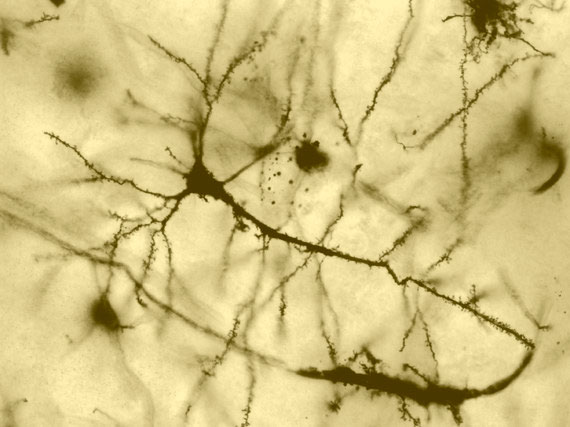
Image: Wikimedia Commons: MethoxyRoxy, Color adaptation: Bernstein Network
/LMU/ We humans can feel, learn and perform purposeful acts only because the sensory information we receive from the environment is translated into nerve impulses, which are then processed in the brain. To accomplish this task, a single nerve cell in the cerebral cortex, to take only one example, may receive and process signals from thousands of other neurons. “In order to understand brain functions, it is absolutely vital to understand how nerve cells integrate these inputs,” says Andreas Herz, Professor of Computational Neuroscience at LMU and a member of the Bernstein Center in Munich. He and his research group have now developed and applied a new analysis tool to characterize the computations carried out by individual neurons. In a new study published in PLoS Computational Biology, they report that the signal processing strongly depends on which part of the cell the synaptic input is delivered.
An illuminating change of perspective
The specific mathematical operation carried out by a nerve cell is strongly influenced by the geometry and the biophysical properties of what is known as its dendritic tree. Dendrites are short cellular processes that extend from the nerve-cell body like the branches of a tree and harbor the functional contacts – known as synapses – that receive signals from the axonal fibers of other nerve cells. Synaptic inputs are first integrated within each individual dendrite and are then combined with the results of other local computations, which together determine the response of the receiving neuron. “To analyze such complex processing cascades, researchers must activate multiple synaptic inputs simultaneously,” as Stefan Häusler, who led the new study, points out. So far, this type of analysis has usually been done, both in the context of experimental manipulations and computer simulations, by monitoring how the cell’s output changes as the inputs are varied.
As a consequence of the complexity of information processing implemented by dendritic trees, it can be extremely challenging to work out which of the myriad steps involved is actually responsible for the observed change in output. “In spite of their fundamental importance for brain function, we are still a very long way from a real understanding of dendritic processing,” says Herz. But he and his colleagues have now developed a novel and promising approach to the problem. Instead of analyzing how the cell’s reaction changes when the input stimuli are altered, the new strategy asks what it takes to keep the cellular response constant. “This enables us to gain valuable insights into how the various inputs interact with each other,” says Florian Eberhardt, first author of the new paper: For example, if the effect of a linear increase in the intensity of one input stimulus can be counteracted by a corresponding reduction in the amplitude of the input to a different dendrite – such that the cell’s response remains unchanged – one can conclude that the two signals are effectively added together in the dendritic tree. If, on the other hand, the relative changes must be balanced to achieve the same effect, the dendritic arbor is inferred to have performed a multiplication of the two signals. In this way, the new ‘iso-response method’ permits one to tease out individual steps in the computation in spite of the enormous complexity of the dendritic tree as a whole.
“We have used the method in computer simulations of pyramidal cells – the most common cell type in the cerebral cortex – and shown that certain types of dendritic processes operate exclusively in a feed-forward mode: They simply transmit the incoming signal in one direction, without any feedback whatsoever,” says Häusler. “This is typically the case when the dendrites are located close to the cell body, where the nucleus resides. Tufts of dendrites that are further away from the cell body exhibit more complex behaviors, which is indicative of feedback effects.”
“So far, we have focused on an established computer model of a pyramidal cell. Our results suggest that different types of dendrites carry out different computations depending on where on the cell they are located,” says Herz. “In the next step, we want to combine our analytical approach with experiments, in order to unveil the dendritic calculations made by real, biological neurons. That will enable us to systematically improve our understanding of the dynamics of informational processing in the brain.”
>> original press release (German text)
Publication
Tuft dendrites of pyramidal neurons operate as feedback-modulated functional subunits
Florian Eberhardt, Andreas V.M. Herz, Stefan Häusler. PLoS Computational Biology 2019